How engineers test Moon landers on Earth
Until a spacecraft is aloft, engineers never really know if their testing regime was appropriate.
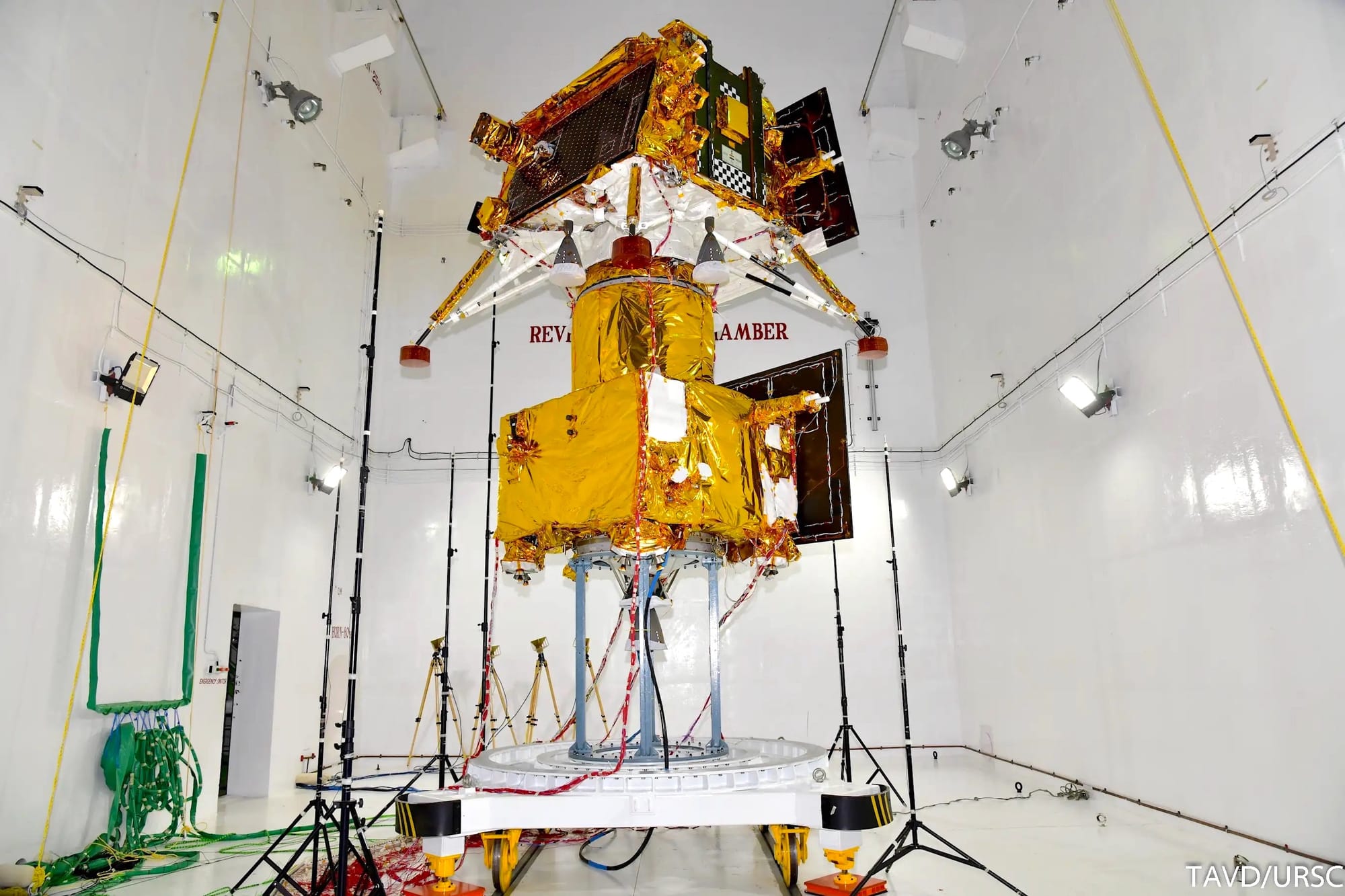
As part of an unprecedented return to our Moon, space agencies, companies, and enthusiasts around the world hope for this decade to mark a string of successful robotic lunar landings. While our lunar ambitions may have risen, having robotic landers fully autonomously orchestrate the firing of their engines from lunar orbit to touchdown remains a daunting challenge. The roughly three-second delay in two-way communications makes it impossible for earthbound engineers to reliably guide lunar landings.
Four out of the eight lunar landing attempts within the past five years have failed; namely Israel’s Beresheet, India’s Chandrayaan 2, Japan’s Hakuto-R, and Russia’s Luna 25. They highlight the fact that while we can adequately test certain things before launching a lander to the Moon, there are aspects we can’t—which is where the uncertainties lie. Let’s take a look at some key tests and challenges in building a lunar lander.
Aside: Lunar lander tests often aren’t conducted with final mission hardware to avoid damaging delicate parts or intricate functions, subsequently causing mission delays and cost overruns. This risk is often worked around by building representative models of spacecraft or their parts for testing.
Enduring the loads
Like every spacebound craft, lunar landers are tested in acoustic chambers to ensure avoiding mechanical damage from the intense, sustained vibrations and sounds of a rocket launch. Additionally, lunar landers test for loads that could be imparted on touchdown. For example, ISRO dropped the Chandrayaan 3 lander’s legs on terrestrial test beds made of simulated lunar soil to ensure that the lander could tolerate a high vertical velocity of three meters per second.
Likewise, US-based private company Firefly Aerospace conducted nearly 100 drop tests on lunar soil simulants and sand to qualify the legs for their upcoming Blue Ghost lander. Firefly aims to carry 10 NASA payloads to the Moon in late 2024 as part of the agency’s Commercial Lunar Payload Services (CLPS) program. “We even tested leg drops on concrete because it’s harder than anything we’ll land on,” says William Coogan, Firefly’s chief lunar lander engineer.
Preparing to be in space and at Luna
To avoid fractures due to swift temperature swings in lunar orbit, every lander spends days or even weeks in thermovac chambers to ensure structural integrity. The chambers achieve a space-and-Moon-like vacuum, simulate its temperature swings, and even replicate unfiltered sunlight using powerful xenon lamps and mirrors. There’s also the concern of radiation damage to electronics, for which landers often host computers and avionic systems made of extensively tested radiation-hardened components.
Protecting lunar landers from the harsh space environment is only part of the story though. We also need to ensure the various hardware and software elements comprising a lunar lander function together as expected.
Kalpana Kalahasti, Associate Project Director of Chandrayaan 3, says her team spent the bulk of the mission’s development time coming up with and overseeing comprehensive ground tests. These included testing landing sensors on a helicopter to mimic different descent phases, examining the performance of engines that must dynamically throttle during descent, and assessing the navigation system’s ability to hover and avoid hazards before touchdown using crane-based setups on Moon-like terrain. “Since testing sensors on aircraft doesn’t simulate hover or low-altitude phases of a lunar landing, we switched to using helicopters for Chandrayaan 3 to better mimic varying altitudes and velocities,” says Kalahasti.
Additional tests can include antenna testing for communications and optical testing for cameras. For NASA’s upcoming VIPER rover mission, which will traverse through rocky terrain at the Moon’s south pole scouting for water ice, the agency drove a model of its wheel in simulated terrain for over 40 kilometers of varying slopes and rock distributions.
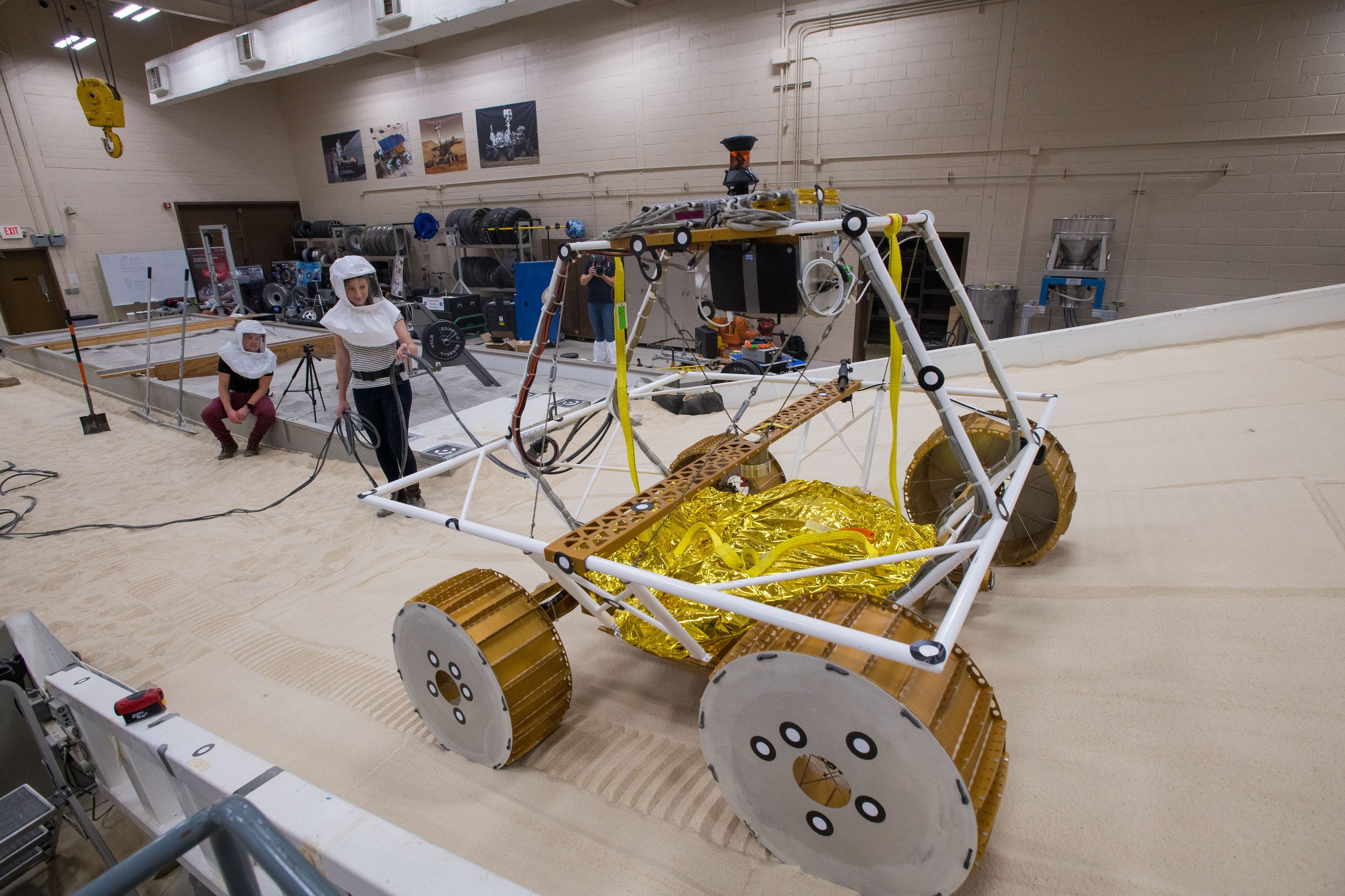
Simulate Moon landings
What you can’t test with hardware, you do in simulations. “Software replicas of your lunar lander help you simulate the lander’s dynamics and its environments,” says Laura Crabtree, CEO and co-founder of Epsilon3, a web-based spacecraft testing and operations platform used by several organizations building lunar landers. To get a better handle on how their lander might behave at the Moon, engineers characterize hardware sensors and put them into simulations, says Coogan.
Teams also simulate key mission phases to identify what kinds of issues their lander can handle by itself, and what needs to be combated via mission control on Earth. “Some real-time data from an ongoing mission is ingested into simulations to test critical commands before sending it to a lander,” adds Crabtree. These methods are sorely needed to meet time-sensitive objectives like putting a lander into lunar orbit, where you often get but one chance.
In fact, simulations are also a great way to make your landing system fail. “We formed a dedicated simulation group to characterize the [Chandrayaan 3] lander’s ability to recover from off-nominal trajectories during descent,” says Kalahasti. The group also sim-tested redundant paths and extreme cases until the landing system failed so as to know its limits, and therefore modify it as needed.
Known unknowns
Some challenges associated with a lunar lander are only apparent when it’s in space, such as the performance of its propulsion system. “You can’t simulate weightlessness in a way that’s indicative of the lander’s response in space. Until you fire a thruster, you will not definitively know the precise force it imparts,” says Crabtree. “So you make a closed loop system monitoring expected versus actual thrust to get you to the right orbits.” Propellent reserves are used to make up for minor deviations in the lander’s actual performance.
Russia’s Luna 25 lander crashed on the Moon during an orbital reduction maneuver on August 19, 2023 due to a 50% longer engine firing than necessary. The Russian space agency’s investigation found that the issue likely stemmed from the software not being designed to prioritize data from the accelerometer, which would’ve otherwise told it that the desired velocity change was achieved well before the engines shutdown.
It’s also hard to pre-determine the safest patch for a lander to touchdown upon. “During the final landing phase, the lander will see new features not present in onboard orbital imagery, including any hazards,” says Coogan. This brings us to a defining feature of modern robotic landers called “vision-based navigation”, wherein a descending lander compares images of the Moon it snaps to preloaded onboard orbital maps to know where it is and accordingly determine where it needs to go. However, tests of such a system on Earth can only represent some Moon-like terrain, and just the final landing phase. This is why Japan’s SLIM spacecraft, which recently landed on the Moon, verified its vision-based navigation ability in lunar orbit prior to beginning its descent.
Private Moonshot challenges
For private companies such as ispace Japan and those part of NASA’s CLPS program, there are additional challenges. They typically cannot invest as much money or time into lander testing as a government space agency. This was partially highlighted on April 25, 2023 with the crash of ispace Japan’s first lunar lander.
As the lander passed over the rim of the Atlas Crater to approach its target landing site within, its laser altimeter correctly reported an increased elevation of about 3 kilometers—corresponding to the crater’s depth. But onboard software designed to keep the lander’s motion stable against certain abrupt values rejected the measurement as erroneous. ispace’s lander, thinking it was closer to the surface than it really was, continued decelerating slowly until it ran out of fuel and fell to a crash. During a media briefing later, ispace CTO Ryo Ujiie said because the company changed the original landing site shortly before launch, the prior simulations testing the lander’s descent weren’t using terrain representative of what the lander faced. Chandrayaan 3 and SLIM sported redundant altimetry systems to ensure safety fallbacks for their landers in such situations.
“Unsuccessful missions can be very expensive to a company. We recognized that and intentionally bid a cost in our CLPS proposal to make sure we had the resources to be successful,” says Coogan.
Ultimately, the fact of the matter is unlike sending spacecraft to Earth orbit, the Moon remains a much more unforgiving destination. Here’s hoping the next set of landing attempts fare better and help sustain the worldwide impetus to return to our Moon.
Originally published on Nature.
Related articles I’ve written previously
- Past mistakes to avoid in our grand return to the Moon this decade
- A brief history of space missions lost to human errors
- Space grade electronics: How NASA Juno survives near Jupiter