How the Apollo missions transformed our understanding of the Moon’s origin
And how going back to the Moon will tell us how Earth and Luna formed.
Where did our Moon come from? The origin of our cosmic neighbor is a fundamental question in planetary science. From Galileo’s first telescopic observations of the Moon to humans walking on its surface, our understanding of its origins has come a long way. Yet it’s far from complete.
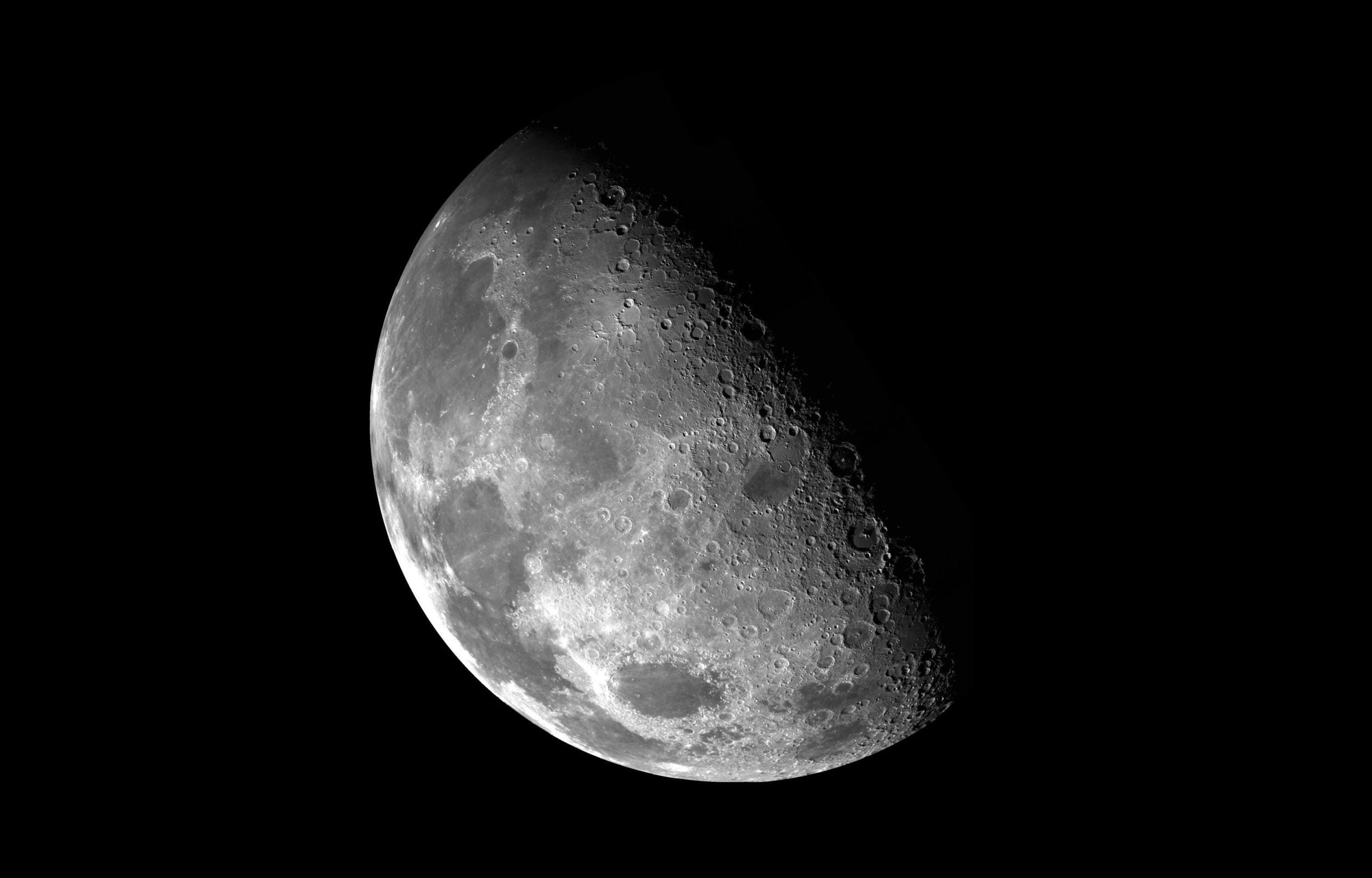
Over the decades, scientists have proposed multiple hypotheses to attempt explaining how the Moon came to be. For this article, I’ll focus on the one with the highest consensus among scientists—the Giant Impact Hypothesis (GIH).
According to the GIH, a titanic collision took place 4.5 billion years ago, when the planets had just formed. A young Mars-sized planet, named Theia, collided with the early Earth. The impact ejected a colossal amount of material. While some of this material escaped to space, the rest stayed in orbit and consolidated to form the Moon.
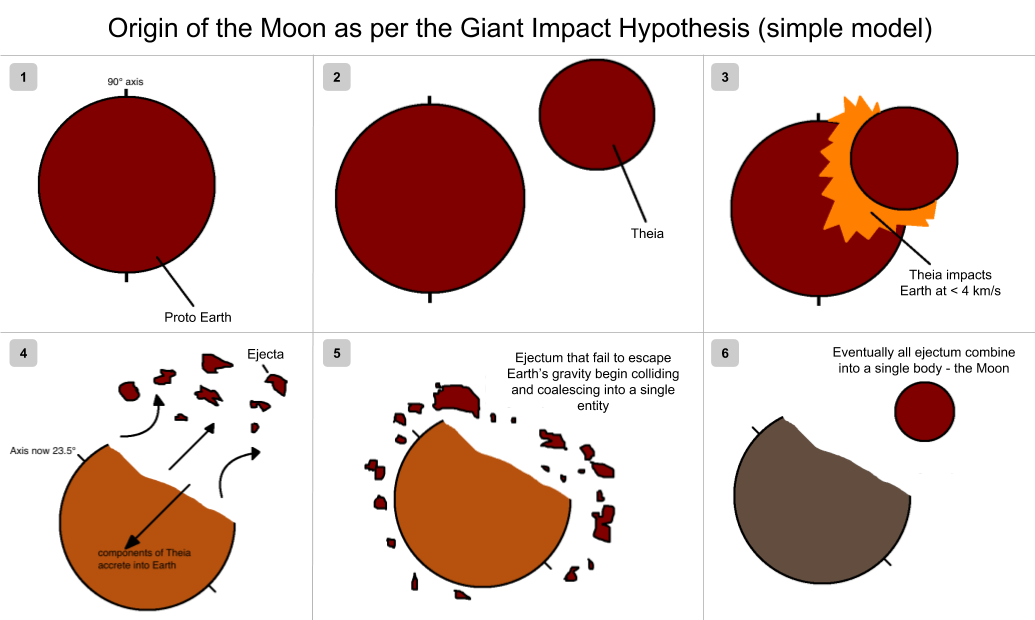
The best evidences for the GIH come from NASA’s Apollo Moon landings between 1969 and 1972, whose 382 kilograms of lunar rock, soil, and core samples brought to Earth allowed us to test multiple hypotheses in varied ways. The picture of the Moon’s formation emerging from Apollo sample studies has been anything but simple. The results have been difficult to reconcile and interpret for scientists. Here’s a rundown of some of the key findings from Apollo.
Findings in support of the GIH
Magma ocean
An implication of the GIH is the newly formed Moon post-Earth-impact must be molten. Scientists estimate it to have been covered in a vast magma ocean at least 500 kilometers deep. With time, this magma would crystallize to form the Moon’s mantle and crust. As this magma crystallized, the heavier minerals, like olivine and pyroxene, sunk to form the mantle. The lower density minerals, principally plagioclase, would float on top, crystallizing about hundred million years later to form a light anorthosite-based crust, best visible to us today as the bright regions on the Moon.
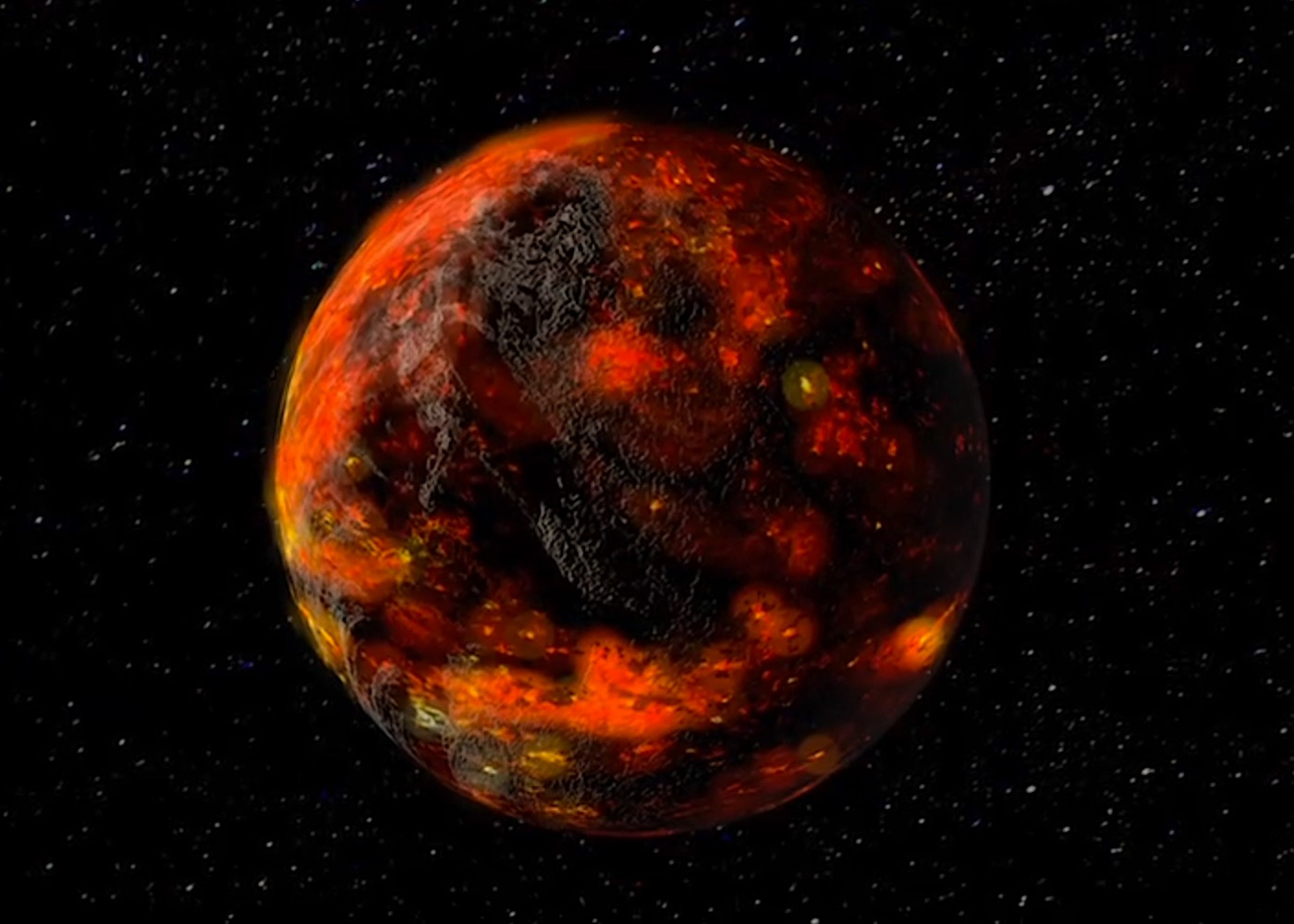
The GIH predicts that during this process, any chemically incompatible elements in the magma would get sandwiched between the crust and mantle, forming what is known as KREEP-rich magma. KREEP is an acronym built from the letters K (Potassium), REE (rare-earth elements) and P (Phosphorus).
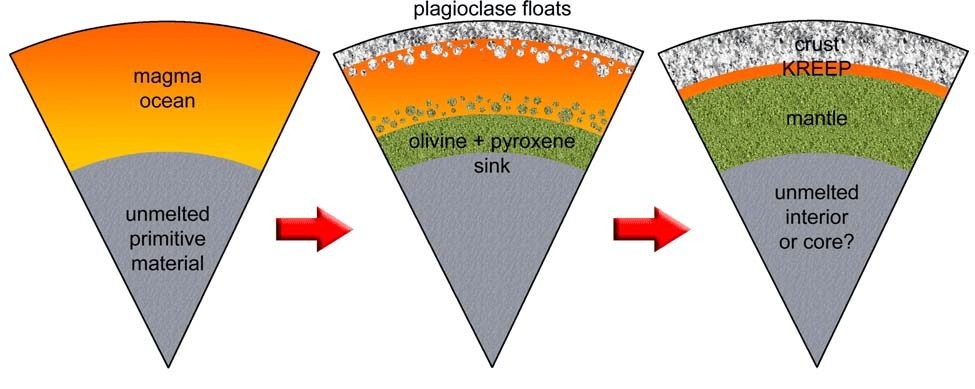
The samples brought to Earth by Apollo 11 contained millimetric fragments from the rocky highlands nearby, the latter of which are part of the aforementioned bright crust. Those fragments were not only found to be made of anorthosites but had KREEP-rich materials too, all but confirming the molten state of the ancient Moon and supporting the GIH.
The lunar core
Also supporting the GIH were three independent experiments on Apollo missions that gave insights into the Moon’s interior. Data from the Passive Seismic Experiments, Laser Ranging Retroreflectors, and Lunar Surface Magnetometers all implied that the Moon’s core shouldn’t be larger than 900 kilometers. That means the lunar core, solid and liquid combined, represents only about 25 percent of the Moon’s radius, in contrast to about 50 percent for other terrestrial bodies like Earth.
The GIH predicts that the Earth-Theia collision would result in Theia’s core getting absorbed into Earth’s core, leaving a smaller core for the Moon. The calculated size of the lunar core from these three independent experiments thus strongly agree with the GIH. It’s worth noting that Earth is the densest planet in the Solar System, which could be explained by the absorption of Theia’s core.
Volatile depletion
Another area of interest is volatiles, which are elements with low boiling points such as nitrogen, water, carbon dioxide, hydrogen and more. Their low boiling points mean their abundance on planetary bodies depletes with time. Large-scale volatile depletion on the Moon could’ve been due to two processes taking place at different times. One is the evaporation of volatiles when the Moon formed, and the other is evaporation by ancient volcanism.
Studying isotopes—different subatomic forms of the same element—in existing lunar volatiles can help us know the Moon’s past. Since isotopes in volatiles deplete differently based on the nature of the event, they can tell us which and how much of the above two processes shaped what we see now. Lighter isotopes of Zinc, for example, would get evaporated in large amounts during the Moon’s fiery formation but remain unaffected by volcanic processes.
Scientists observed in Apollo samples that the lighter isotopes of Zinc are less abundant than the heavier ones. This means large amounts of volatiles were indeed lost during the Moon’s formation, supporting the GIH prediction of a Moon being volatile-rich.
Findings against the GIH
Titanium isotopes
If the Moon indeed formed from the collision of two objects as the GIH posits, it should’ve inherited some of its material from Earth and some from Theia. One way to test that is to measure the titanium isotopic composition of the Moon and compare it to Earth’s. If the Moon formed from both Earth’s and Theia’s materials, its titanium signature should be a mix of both. Titanium is a good element to measure because it isn’t easily vaporized and tends to remain solid or molten even under high heat. Meaning, titanium should roughly be in the same state now as it was during the Moon’s formation.
The comparative analysis of titanium in Apollo samples indicates that the Moon’s titanium came from Earth alone and is not a mix of Earth and Theia. In stark contrast, meteorites found on Earth contain large variations in titanium isotopes, indicating their distinct and varied origins. How then could the GIH be correct?
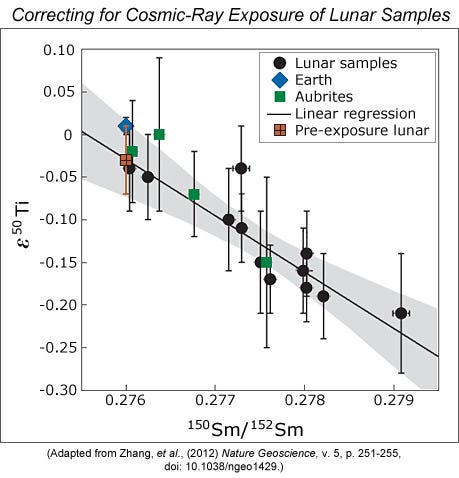
One unlikely explanation is that Theia had the same composition as Earth but that’s a long shot. Computer simulations of the GIH collision by Caltech researchers in 2007 calculated the origin of various materials relative to the Sun, and how they were distributed across the early Solar System. They found the likelihood of Theia having an identical isotopic composition to Earth to be less than 1%.
On the other hand, scientists proposed in 2021 that the two continent-sized higher density regions in Earth’s mantle, one beneath Africa and one beneath the Pacific Ocean, could be Theia’s remains. In 2022, scientists published a study of six lunar meteorites, which concluded that the Moon’s noble gases like helium and neon come from Earth.
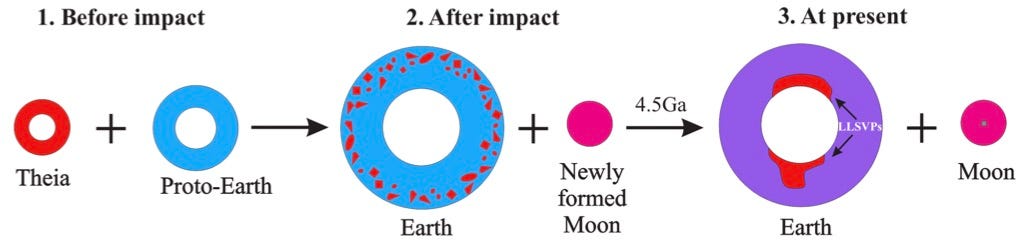
A shared water source for the Earth and the Moon
Much like the case with titanium, the isotopic compositions of lunar hydrogen and oxygen can be compared with that of Earth. The volcanic glass samples brought back from Apollo 15 and 17 had minor quantities of water in them. The isotopic composition of hydrogen in water in them was nearly identical to that of water in Earth’s mantle. Likewise, the Moon’s oxygen isotopic composition, measured from Apollo 11, 12, 15, 16 and 17 samples, also show identical natures to Earth. When compared to other Solar System objects, like Mars, the Earth-Moon system is thus compositionally distinct and identical.
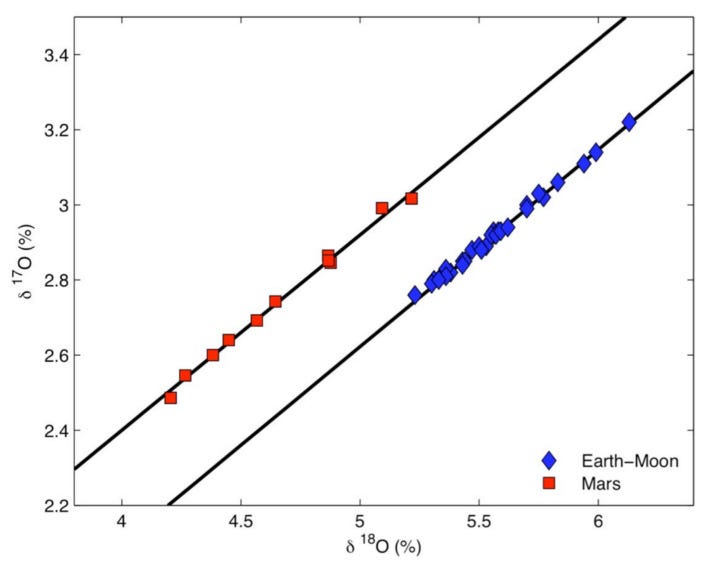
These results indicate that the process that formed the Moon involved objects that were created in this neighborhood of the Solar System, and that both Earth and the Moon shared the same water source. The nature of water in the Moon’s interior is thus not compatible with the GIH.
What’s next?
While various other modifications have been proposed to the GIH to account for both the evidence and counter-evidence, the fact remains that the Moon’s origin is very much a mystery. Despite Apollo missions landing in largely similar geological areas, they completely turned our understanding of the Moon’s origins on its head. At the same time, its results left us with more knowledge but farther away from a conclusion.
To continue piecing together the complex origin and history of the Earth-Moon system, we need samples from new locations, including geologically pristine rocks below the lunar surface and from the farside. We need to probe the nature of water on the Moon’s poles and in the lunar interior to know their origins. If the Moon really formed from the debris of a collision between early Earth and Theia, many scientists think the impact’s intense heat makes it unlikely for the Moon to have gotten or retained much water or its constituents while coalescing.
A nice, detailed review paper published in 2021, Origin of the Moon, summarized recent advances in our knowledge of the same. The paper highlights ten key datasets that would clear the dust on our Moon’s formation. This includes getting a better understanding of the role of magnetic fields, which likely accelerated the process. An unintuitive inclusion in the list is measuring the isotopic composition of Venus so that we can get a better hold on what made the inner solar nebula, and thus Theia.
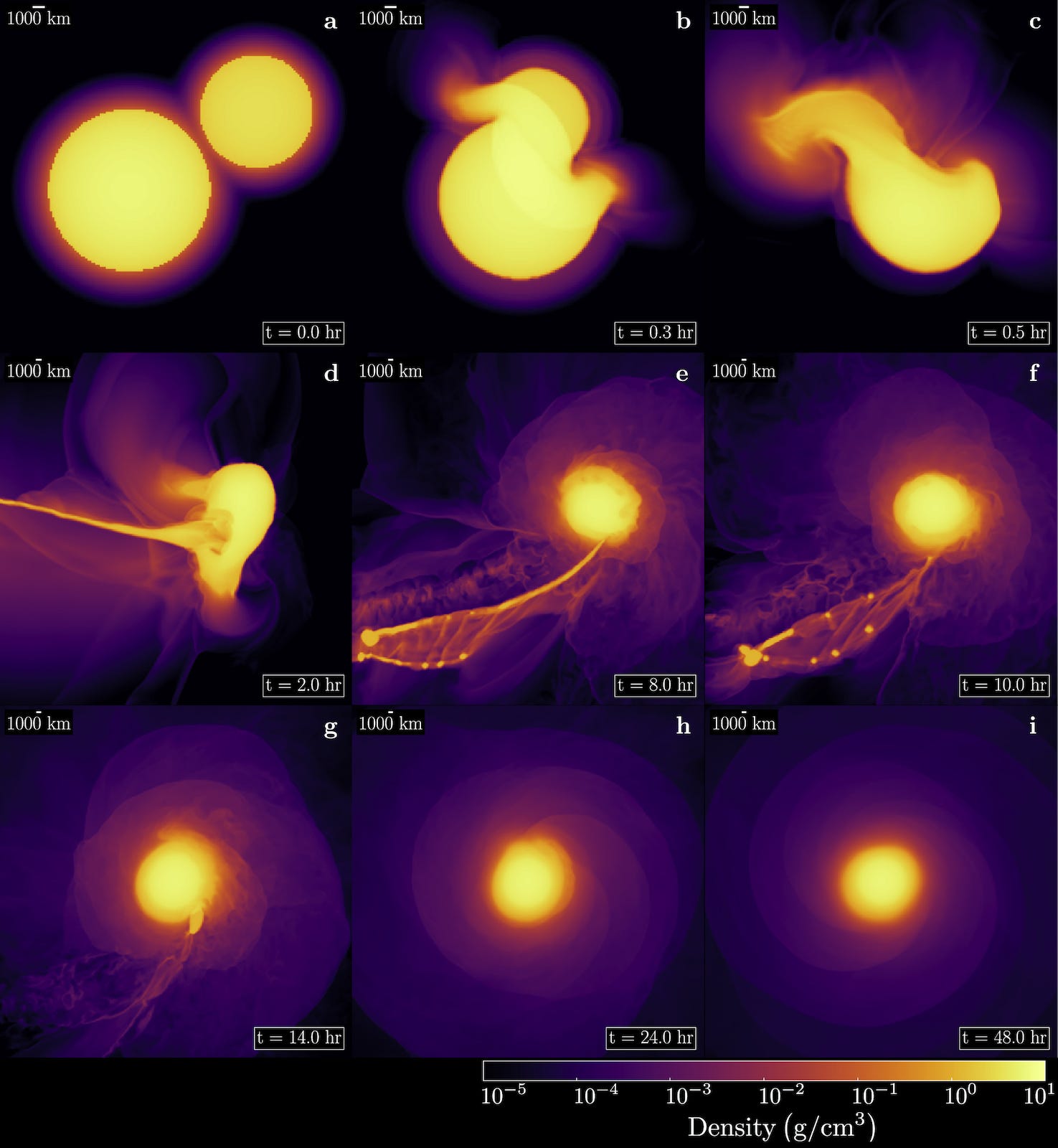
This is where NASA’s Artemis program is most intriguing. Artemis is expected to offer abundant opportunities to bring samples from the Moon, particularly from some pristine locations on the south pole. Near-term missions like China’s Chang’e 6 will also collect samples from the Moon’s south pole, possibly from the highly desired South Pole-Aitken basin. With robotic instruments for surface-only studies becoming increasingly advanced though, scientists suggested in 2021 that even missions like those under NASA’s CLPS program can lend significant insights by focusing on precisely measuring trace elements, especially in key and diverse places on the farside.
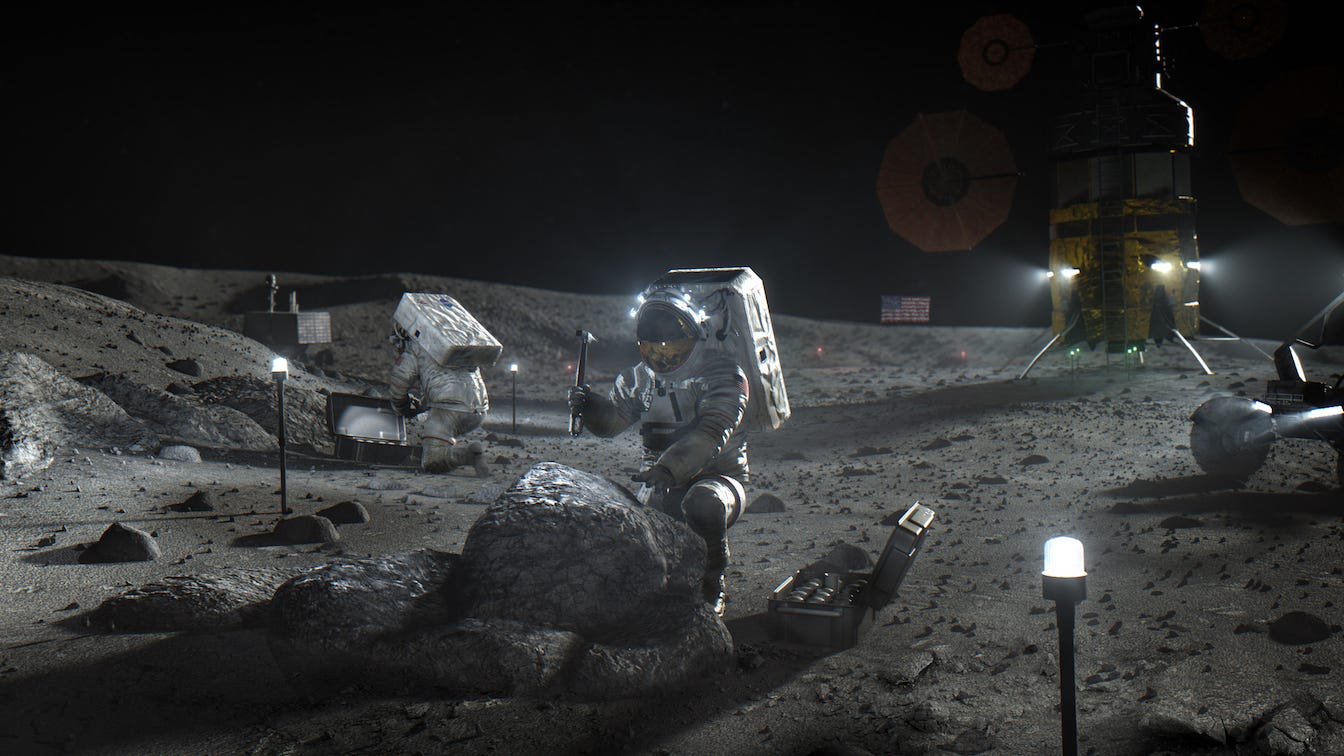
What a profound success it would be to identify the origin of our dear Moon. For now, it remains a mystery. Here’s hoping that the renewed and global frenzy of going to the Moon sticks around this time, as there are plenty of reasons to explore Luna.
Originally published in 2018, significantly updated and rewritten in September 2022 to include new findings and reflect the new mission landscape.
Thanks to Phil Stooke from University of Western Ontario for having fact-checked the original version of this article.
The original article was republished by The Planetary Society, and featured as part of its special coverage for the 50th Apollo anniversary.
→ Browse the Blog | About | Donate ♡