How NASA decides where to land on Mars
The multi-decade drive to explore the question “Did Mars once have life?” has opened the way for future pinpoint landings throughout the solar system.
NASA’s successful touchdown of its $2.7 billion Perseverance rover in Mars’ Jezero crater on February 18 is a triumphant culmination of four decades of increasingly challenging landings on the Red Planet. The now dried-up river delta and lakebed of Jezero is so ideal to sniff out signs of fossilized creatures from the planet’s warmer, wetter, more habitable past, one might wonder why NASA’s number of prior missions never visited here. The answer is simple: Attempting a landing in such complex terrain has been impossible until now.
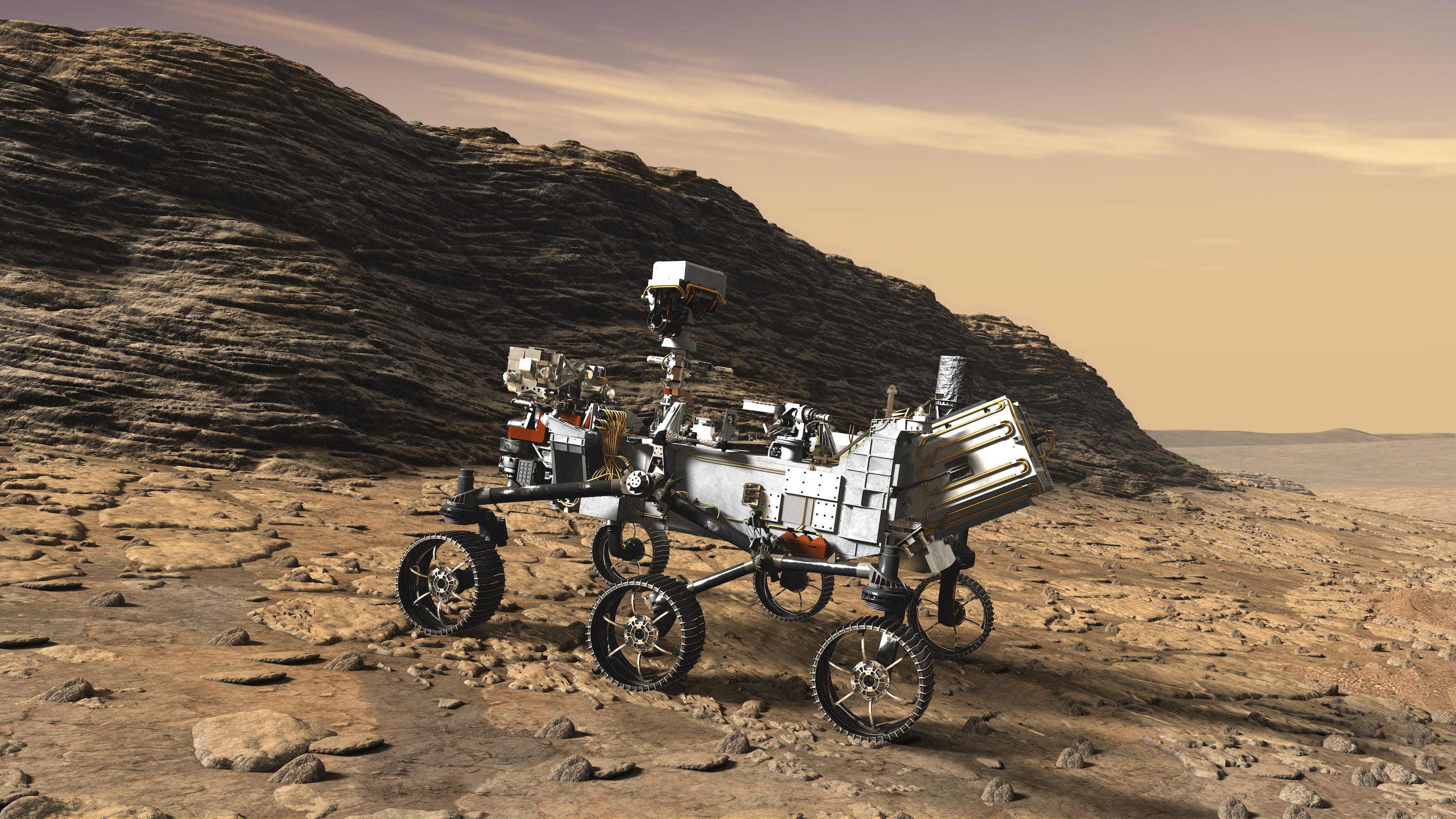
When we begun exploring Mars with robots in the 1970s, the best available pictures of the planet’s surface were so crude that targeting where to land was akin to playing a blindfolded game of darts. NASA’s first landing efforts, the Viking 1 and 2 missions of 1976, had to snap images from orbit before mission planners could pick landing sites, and even then any semblance of safety was far from guaranteed. The vintage tech at the time could only ensure that each lander would come to rest somewhere within an ellipse 300 kilometers long and 100 kilometers wide, hopefully close to a central point scientists had flagged for investigation. Ultimately, NASA officials put this “landing ellipse” on the safest places they could see from orbit, setting down each Viking in a smooth, near-featureless plain stretching more than a thousand kilometers.
Shrinking the ellipse
By the time Spirit and Opportunity, NASA’s twin Mars Exploration Rovers (MERs), were to land in 2004, the space agency’s engineers had learned how to shrink the ellipse to 150-by-20 kilometers. This tenfold improvement over the Vikings was possible thanks to better understanding of Mars’ atmosphere as well as new techniques for spacecraft navigation. By this time, NASA also had much better map and mineralogy data from its orbiters. Scientists used that information to have Opportunity target a region where there was hematite, a mineral that only forms in the presence of water, as a way to study an ancient, alien watery environment.
“Combining landforms and mineralogy data of a region, and taking advantage of engineering upgrades, Opportunity was the window into the future of how we choose landing sites,” says Briony Horgan, a co-investigator on the Perseverance mission.
NASA had considered landing the MER missions in a much richer site, Gale Crater, where the agency’s Curiosity rover ultimately landed in 2012 and still operates today. But the crater’s central mountain—near which Curiosity found evidence for ancient, microbe-friendly freshwater lakes—was itself a landing hazard for the MERs. The skinny landing ellipses of MERs were as long as the crater itself, posing a real risk of the multi-hundred-million-dollar rovers slamming into the mountain or crater walls during their descent. So NASA engineers gave their successor, Curiosity, the ability to steer to compensate for large deviations that could be caused by wind, shrinking the landing ellipse to an impressive 20-by-7 kilometers.
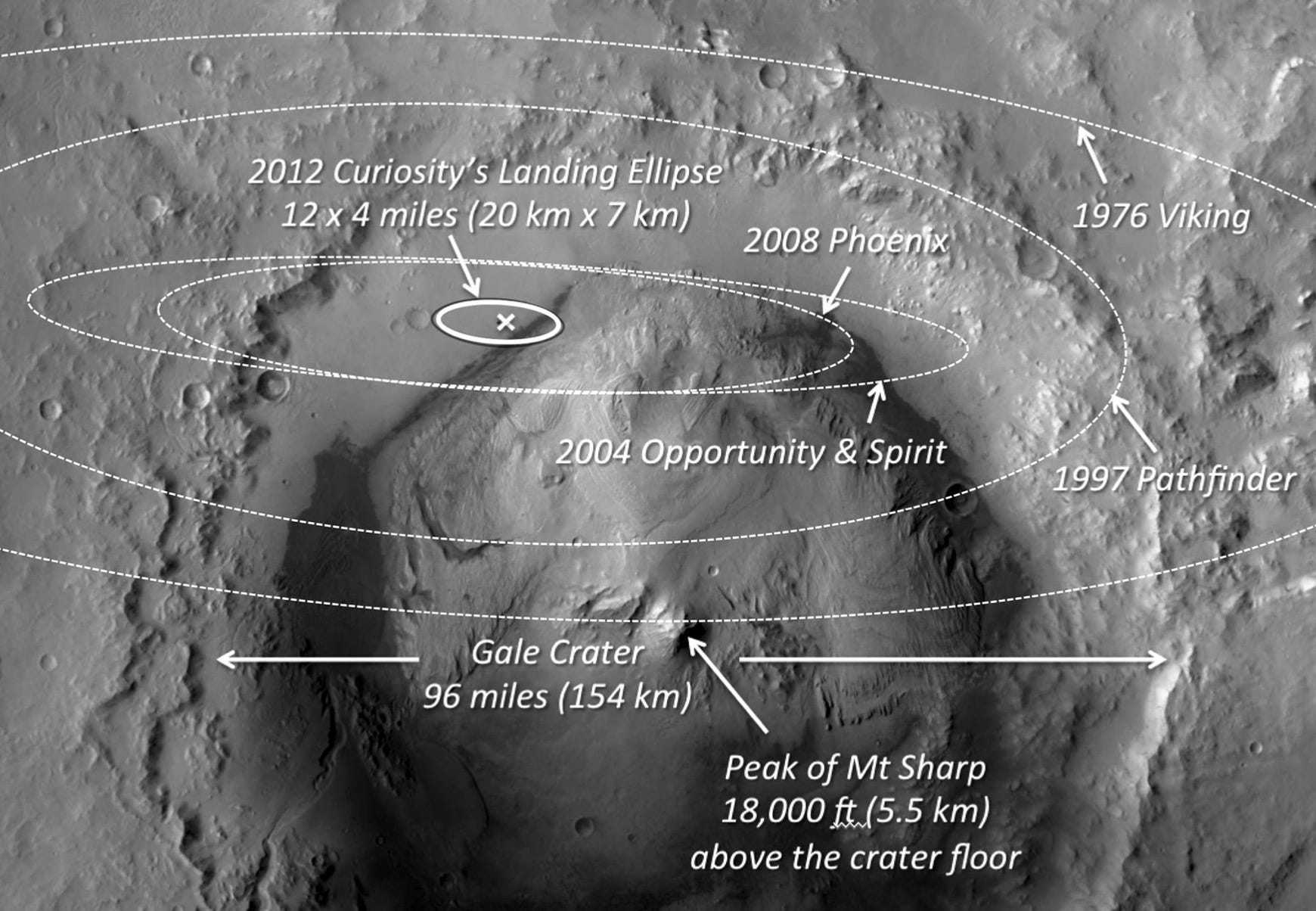
Thanks to its nuclear power supply, Curiosity could also drive much longer distances than the MERs, and had a mission life span of years as opposed to a few months. This was critical to Gale’s selection as Curiosity’s landing site since engineers could now drive the rover to the mountain after a safe landing away from it. “Even if a MER rover had safely landed inside Gale, not only would the mission have been much different but neither of them could’ve gone to the mountain,” says Melissa Rice, a collaborator on MERs, and a co-investigator for the Mastcam-Z camera on Perseverance.
Nestling into Jezero
For Perseverance, Curiosity’s previous success allowed far more landing sites to be considered. NASA’s latest rover is a near-clone of Curiosity and used the same stunning “sky crane” method for its touchdown. Data from Curiosity’s Entry, Descent and Landing (EDL) helped engineers reduce Perseverance’s landing ellipse by about 50 percent, says Chloe Sackier, an EDL systems engineer for Perseverance. “While EDL for Perseverance looks similar to Curiosity, it’s a lot smarter on the inside,” she says. “We have a new technology called Range Trigger to deploy the supersonic parachute based on where the spacecraft thinks it is, as opposed to deploying at a predetermined velocity, which led to Curiosity flying slightly longer in the Martian atmosphere.”
After prior missions painted the picture of a watery, habitable early Mars, NASA wanted Perseverance to actively seek signs of past life. Mission planners had much more satellite data to play with too. “Some sites that were first proposed for Curiosity became serious contenders for Perseverance’s selection after the Mars Reconnaissance Orbiter revealed more about the minerals and rock types at those sites,” Rice says.
Armed with high-resolution imagery as good as 30 centimeters per pixel and detailed mineral maps, more than 150 scientists carefully examined in excess of 60 promising locations, filtering them over five years and ranking the best ones. In the 2017 grand finale filter of this habitability- and geology-flexing competition, 3 sites rose to the top.
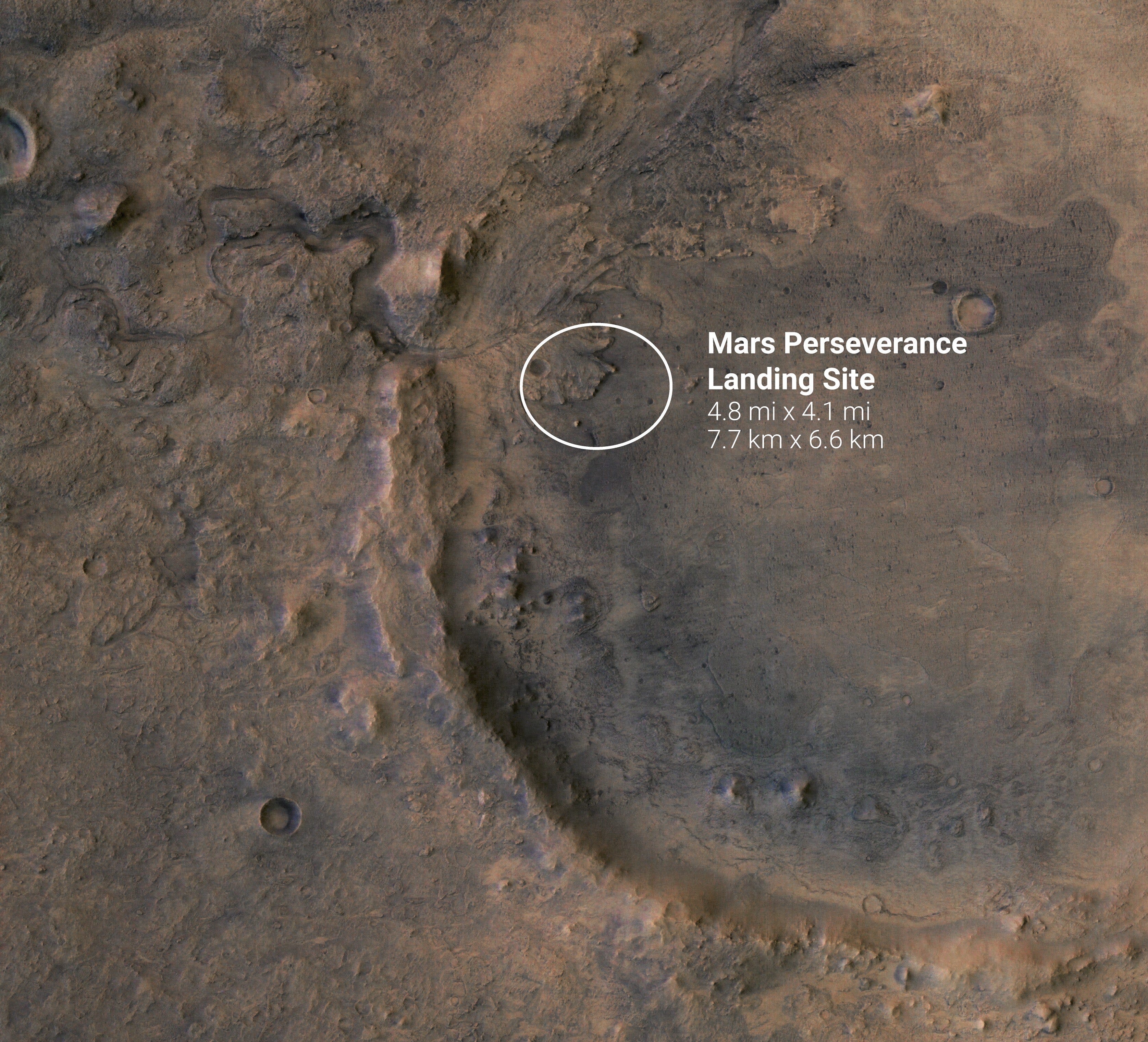
Although all three sites represented potentially habitable ancient locales, Jezero won out because its lake-delta system uniquely offered multiple, distinct opportunities to probe for signs of past life. “Jezero might be most well-preserved ancient delta, representing the most habitable period in Mars history when we see evidence for rivers flowing all over the planet’s southern hemisphere,” Horgan says.
However, Jezero’s delta could not be efficiently targeted even with the improvements made on Curiosity’s landing ellipse. The delta’s most promising feature—its sprawling, sediment-rich base—was a landing hazard due to adjacent tall, wide cliffs. At best, Perseverance could land east of the delta and spend months driving to the base.
At least that was the case until 2019, when NASA revealed that Perseverance would use a new technology called “Terrain Relative Navigation (TRN)”. Instead of descending on Mars with a blind eye, a spacecraft with TRN can look at terrain features below it and compare it to preloaded maps to know where it is, allowing it to autonomously and flexibly guide itself to a more pinpoint landing. With these advances, the Perseverance landing ellipse was now a solid 7.7-by-6.6 kilometers.
The introduction of TRN completely changed the game for scientists. “TRN allowed nestling into landing areas almost anywhere we wanted,” Rice says. “Landing right at or near the preserved Jezero delta base, where the rivers deposited concentrated organic material, was possible. TRN made the mission land-on rather than go-to.”
“We historically had to look for large, smooth ‘parking lots’ for landing things on Mars,” Sackier says. “We could now open up sites like Jezero due mostly to the inclusion of TRN, which allowed us to target multiple little parking lots within the landing ellipse instead of a giant one.”
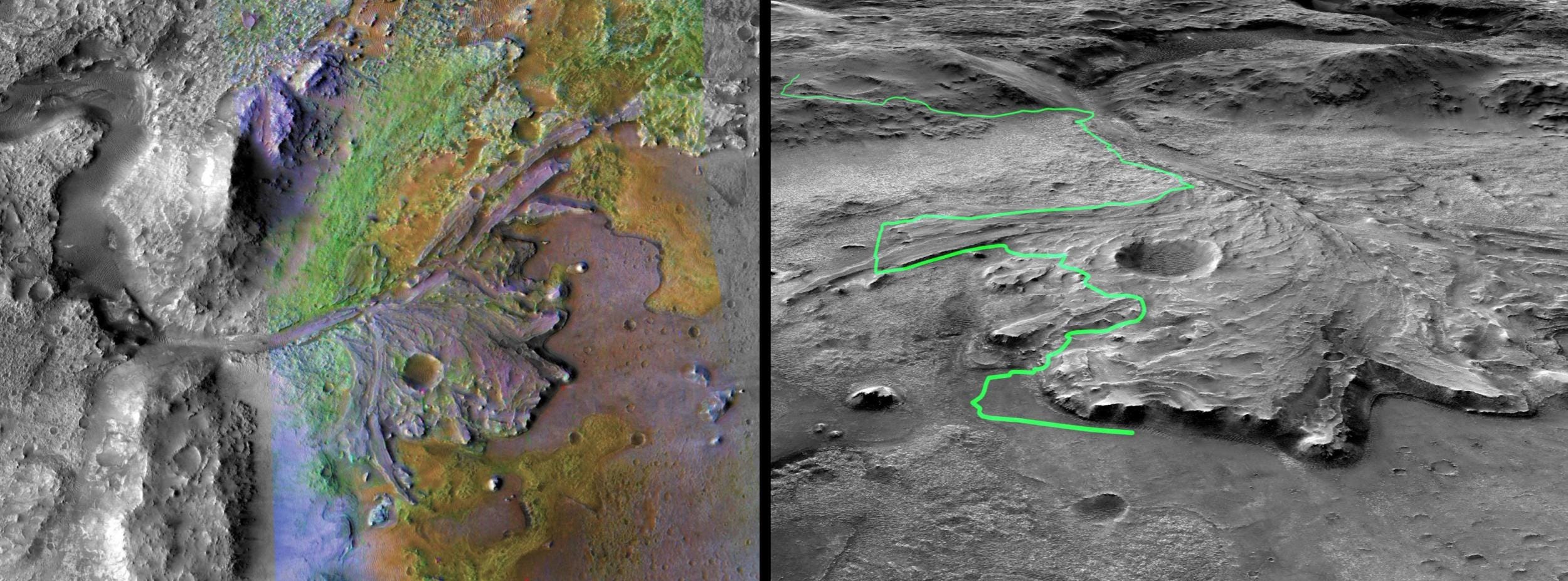
Sure enough, Perseverance’s landing system performed remarkably well on February 18, 2021 as it landed the rover in a smooth little parking lot within an otherwise rough terrain just 1.7 kilometers away from the center of the landing ellipse. Perseverance will spend the next 2-3 years traveling across the delta’s dry riverbeds to the edge of Jezero crater, searching for various biosignatures. It will gather and store samples of its most promising finds, depositing some of them for eventual retrieval by a ‘fetch rover’ from the joint NASA-ESA sample return mission launching no earlier than 2026. Engineers will use data from Perseverance’s EDL to further tighten the landing ellipse for the fetch rover, hopefully shrinking the distance it needs to travel across the planet’s surface.
Where to next?
Even with these impressive advances, some sites on Mars are still out of reach for spacecraft landings and will likely remain so for the foreseeable future. For instance, scientists cannot propose landing on high-altitude features such as Olympus Mons because the atmosphere overhead is too tenuous to sufficiently slow down a spacecraft. Regions with very rough terrain or steep slopes are also off-limits, even with TRN. Furthermore, features such as polar ice caps, canyons, lava tubes and sand dunes offer poor prospects for wheeled rovers, demanding alternate forms of mobility.
Nevertheless, the precision landing technologies NASA has developed for Mars over decades will enable exploring uncharted places in the solar system. “TRN, for instance, can enable landing a spacecraft on Jupiter’s moon Europa while avoiding its huge icy cliffs, boulders, and other obstacles,” Rice says.
The payoff of NASA’s Mars program is not just technical. “We learned how to efficiently operate a spacecraft on another planet for long periods of time. We give Curiosity commands three to four days in advance and it semiautonomously completes them. That experience is enabling ambitious things at the Moon, like having the upcoming VIPER rover, launching in 2023, largely autonomously explore permanently shadowed regions on the lunar poles,” Horgan says.
TRN will also enable NASA’s Artemis robotic and crewed missions to perform precision landings on the Moon’s south pole, which lacks large, flat areas. Moonbases in the future would also benefit from TRN as robotic cargo missions would only need to drive short distances post-landing.
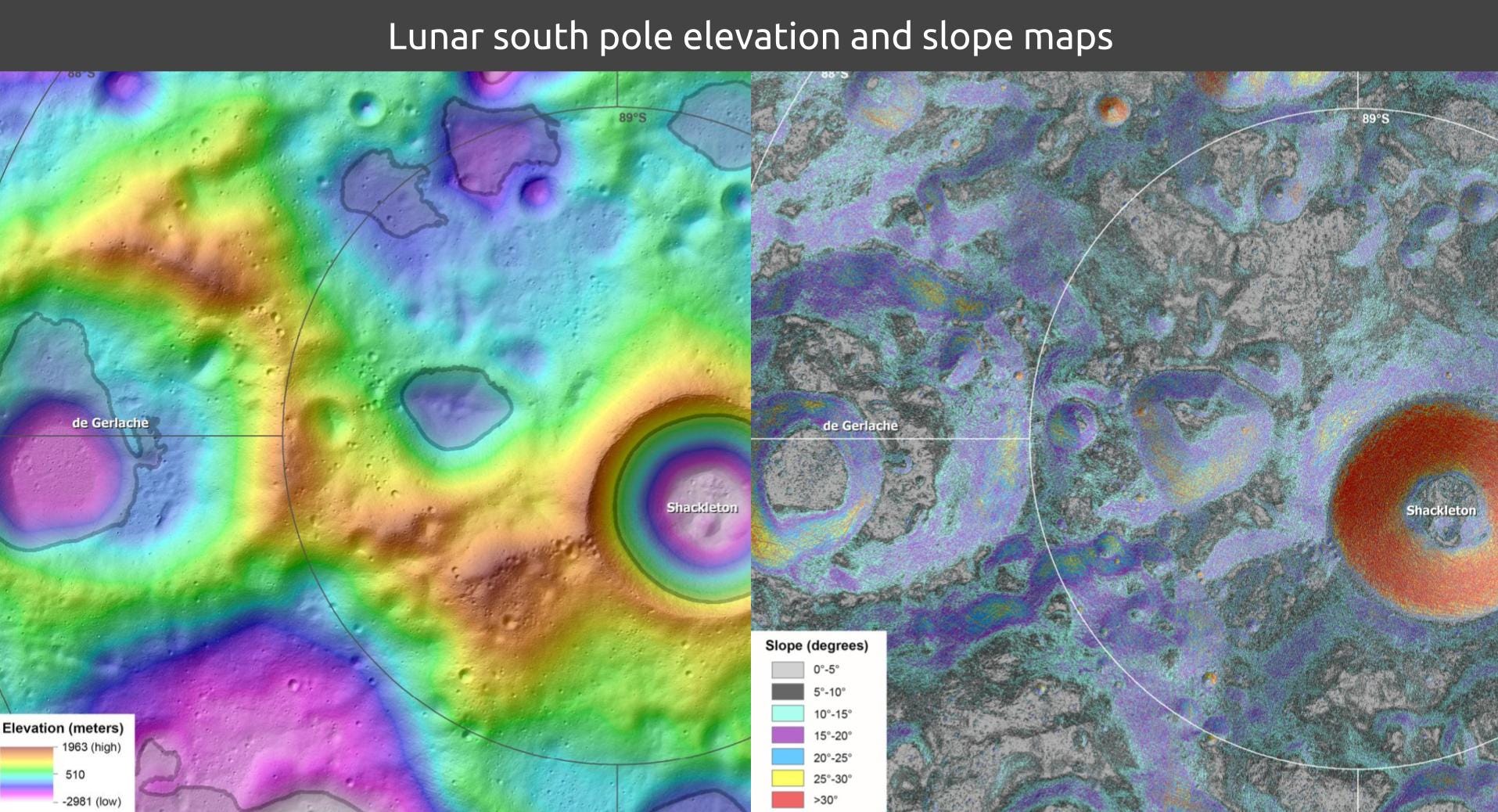
Thanks to NASA’s ambitious, multi-decade Mars endeavors, a new era of pinpoint landings throughout the solar system may be underway.
Originally published at Scientific American.
Like what you read? Support me to keep me going.